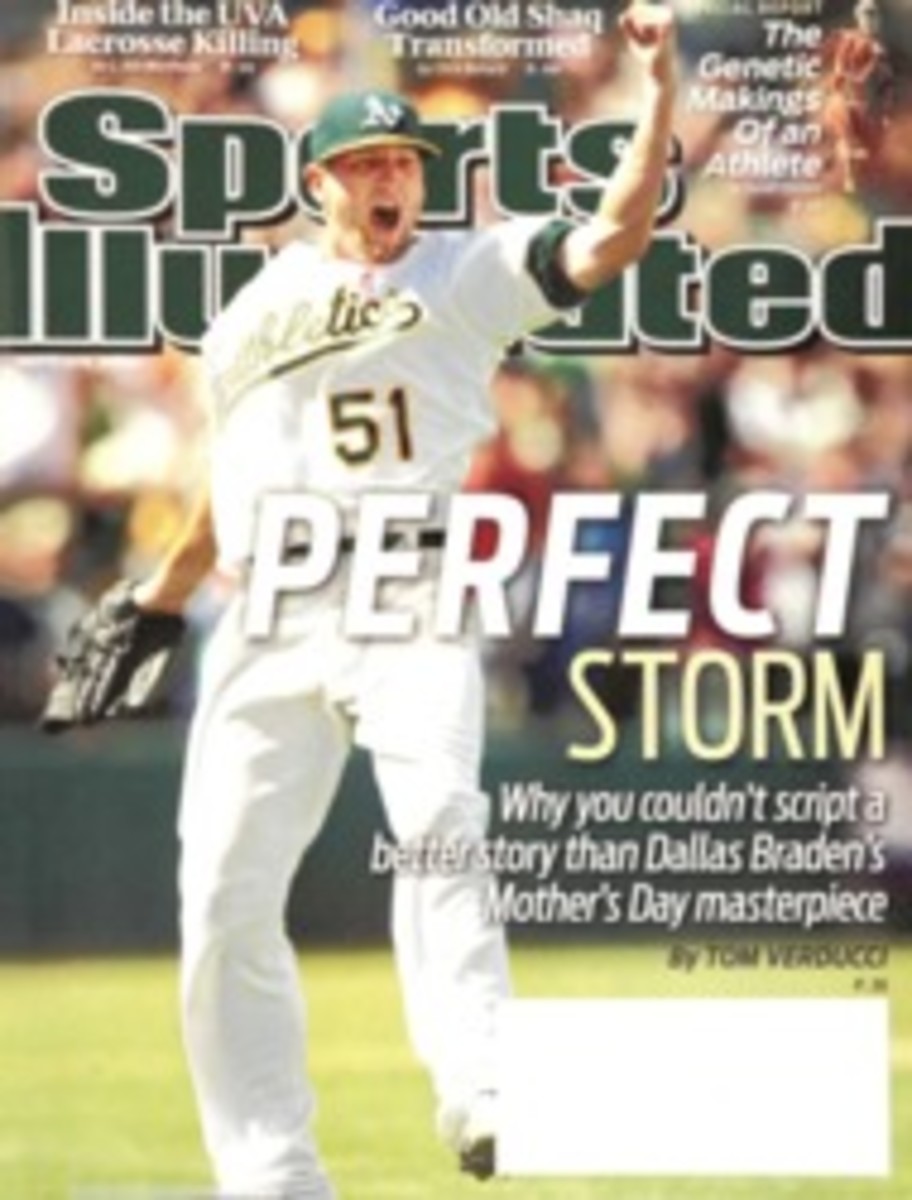
SPORTS GENES
Remember the guy or girl in high school who just had it? He was all-everything at quarterback and high jump; she led the pitching rotation and was also the starting point guard. Naturals. Or were they?
Did Ken Griffey Jr. inherit good genes, or did he become a superstar because he grew up in a clubhouse? Or both? For the price of a family outing at the ballpark, some companies will tell you if you have a certain sports gene. I have the sprinter gene, for instance, and you probably have it too.
So are there really such things as sports genes? If there are, do only elite athletes have them, or do the pros separate themselves from the rest of us more by their work ethic? And the work ethic—is there a gene for that?
There's only one place to look.
THE GENETIC PLAYBOOK
AT THE CENTER of our every cell lies the twisting ladder of the double helix. A mere four molecules—adenine, cytosine, guanine and thymine—pair off three billion times to make up our DNA, the instruction manual for our bodies. Twenty-five thousand sections of each DNA ladder are especially important. They are genes, and it is they that actually tell the body how to build itself.
In April 2003 an international consortium of scientists announced the completion of the Human Genome Project. After 13 years of work (and 200,000 years of modern man), the project had mapped the human genome; all 25,000 regions of DNA that contain genes had been identified. Now researchers knew where to begin looking for the biological foundations of many of our traits, from hair color to hereditary disease to athleticism.
But even if scientists know where the most important parts of the manual are located, they don't necessarily know how to read them. In fact, the precise functions of most genes, including many so-called sports-performance genes, remain mysterious, and thus talk of splicing DNA to create the perfect athlete is a bit premature.
Already, though, scientific research gives us a fuller picture of how we evolved into athletes, and it suggests that some things that appear to be largely genetic (such as East African dominance of distance running) might not be, and that other things that seem entirely voluntary (such as an athlete's will to train) might in fact have an important genetic component.
Scientific studies that associate particular genes with athleticism are published literally every month. These genes exist, in differing versions, in all of us, from All-Pro to average Joe. As the study of performance genes accelerates, more assumptions about sports and genetics will no doubt prove false, and new answers will reshape our view of why and how some people become NFL running backs or Olympic swimmers while others struggle to pass phys ed.
The findings are already raising ethical, social and even economic questions. Yet even as the research explains many of our athletic differences, it may reveal more important biological truths about us as a single humanity.
WE ARE BECAUSE WE RUN
IN OUR GENES we are all distance runners. Let's start at the cusp, just before humans became the earth's marathoners. Two-and-a-half-million years ago, our ancestors lived in the trees of the East African woodland, foraging for fruit and digging up tubers. They were wide-hipped, hunched and hairy, and the giants among them were all of five feet tall. But their world was changing dramatically. Their forest home had begun to give way to hot, dry savanna, with few trees and with grass short enough to give sight lines that stretched until the earth curved away. Our forebears saw for the first time the hordes of wildebeest and antelope that filled the plain.
Gradually these ancient, mostly vegetarian primates dropped from the trees and went looking for steak. Initially they might have used vultures as their guides, racing hyenas to scavenge the leftover brains and bone marrow of dead antelopes. For the first time in history a two-legged mammal had reason to run long. Those who could jog in the punishing equatorial heat could beat the hyenas to a carcass. They could survive another day, perhaps long enough to have children.
The major changes that took hold in the body over the next half-million years were examined in 2004 by biologists Dennis Bramble of the University of Utah and Daniel Lieberman of Harvard. Their conclusion contradicted the common assumption that human running was simply a by-product of walking. Nearly every one of the major anatomical changes en route to modern man, the professors argued, conspired to make him the hot-weather endurance running champion of the savanna.
There is, for instance, the rubbery neck ligament that acts like a shock absorber for the head during running; the glut of sweat glands to help keep the body cool while running; the lack of body fur for the same reason; shoulders that move, unlike in apes, independently from the neck so that the arms can swing while the head remains still; long legs and narrow waists; larger surface areas in hip, knee and ankle joints, again for improved shock absorption; short toes, which are better for pushing off than for grasping tree branches; an arched foot, which acts as a spring; and big butt muscles to keep us upright. "Have you ever looked at an ape? They have no buns," Bramble says. "We think running is one of the most transforming events in human history."
No longer content merely to scavenge, our ancestors, despite having no greater weapons than sticks and stones, became deadly hunters. They overwhelmed their perspirationally challenged quarry with a methodical chase that lasted until the beasts, unable to pant sufficiently while fleeing, simply gave up from heat exhaustion.
Descartes said we are because we think, but consider that we thought only after we ran. Even our large brains developed because we ran, growing only once our endurance enabled us to gorge on animal fat and protein. We are who we are—the only sweating, largely hairless bipedal mammals—because we ran. As Lieberman puts it, "Endurance running is hardwired into our anatomy and physiology."
For decades running was considered an unimportant part of human evolution because we humans are such pathetic wimps at sprinting. In his world-record 200-meter dash, Usain Bolt averaged a little more than 23 mph for nearly 20 seconds. That would make him an abject failure as a savanna hunter because an antelope can double that clip for minutes at a time. But with the help of our upright stance (which exposes less of our bodies to the sun) and our profuse sweating, we can outrun just about any other animal on the planet if the race extends over hours in searing midday heat.
Sound far-fetched? Consider that humans have beaten horses in the 22-mile Man Versus Horse Marathon in Wales, and humans routinely win the 50-mile Man Against Horse Race in Prescott, Ariz. And note that in Southern Africa a small number of San Bushmen, the world's oldest community of modern humans, still hunt by separating an antelope from its pack and chasing it for hours in 105° heat, until the animal simply stops running and waits to be killed. Or note that any Tom, Dick or Oprah can complete a marathon with proper training and sensible pacing.
Granted, most of us are a bit out of practice, but even you who walked the mile in high school gym class have the genetic stuff of an endurance hero. Lieberman suggests that our love of sports is partly an outgrowth of our running past. "Animals play at things that are important to them," he says, "and we play at running."
Yet there are massive differences in ability among individual humans even in running, a simple and global sport in which lack of access to equipment does not inhibit achievement. There is, for instance, an undeniable trend in elite running. The 18 fastest marathon times in history belong to East Africans—Ethiopians or Kenyans—and the top 10 sprinters ever in the 100 meters are men of West African descent. In short, they are all black.
ALL GENES ARE AFRICAN
WE ARE ALL black. Not in the sense that our skin is of a shade that protects against equatorial sunlight, but in the sense that Africa is contained in our every cell.
It starts with our brown-eyed, many-times-great-grandmother, the woman scientists call Mitochondrial Eve. Mitochondrial DNA is a genetic material that is inherited from one's mother, and as it happens, every one of us shares some of it with Mitochondrial Eve, a woman who lived in sub-Saharan Africa around 150,000 years ago, when the entire human population consisted of a few tens of thousands.
Since the mid-1990s scientists have been following the path of mankind's genes away from Mitochondrial Eve by collecting genetic data throughout Africa and beyond. Geneticists Kenneth Kidd of Yale and Sarah Tishkoff of Penn have been among the leaders in this endeavor. Some of their work supports the "recent African origin" model, which suggests that all modern humans can trace their ancestry to a single population in east-central Africa as recently as 100,000 years ago. Since humans branched off from our common ancestor with chimps about six million years ago, that means we're about a one-minute drill out of Africa.
What Kidd, Tishkoff and others have found is that genetic variability—differences in DNA among people—is greater among Africans within a single population than among people from different continents outside Africa. This is because all human genetic information was contained in Africa not so terribly long ago, and our ancestors who left Africa—most likely a single group of no more than a few hundred people—took only a small portion of it with them en route to populating the world. All of us outside Africa are genetic subsets of the subset that left Africa. So despite the fact that black Africans may share certain obvious features, such as dark skin, when it comes to an African's entire genome, there might be more difference between him and his next-door neighbor than between Dirk Nowitzki and Ichiro Suzuki. In fact, the farther a group of native people is from Africa, the less genetically diverse it tends to be. In some sections of DNA, Kidd says, there is more variation within a single African Pygmy population than in the entire rest of the world combined. "In that sense," Kidd says, "I like to say that all Europeans look alike."
This has tremendous implications. In some cases, for example, classifying people solely according to their dark skin will impart no genetically based knowledge about the group's members other than that they have dark skin. Take, for another example, sports. Kidd suggests that for any activity that has a genetic component, the world's most naturally gifted person is likely to be African (or recently removed from Africa, as are African-Americans and Afro-Caribbeans), as is the world's least naturally gifted individual. So both the fastest and slowest runners might well be of recent African descent.
That's not to say that scouts should be looking for the next MJ or Usain among African Pygmies. "There are some anatomical features that would intervene," says Kidd, referring to the Pygmies' short legs, but he adds that "you might find the most naturally gifted basketball players in some of those populations in Africa where height and coordination are on average very high, and where you have a lot of other genetic variation within that group."
Of course, the only real way to test this idea would be to know which genes influence athleticism, and then to look for them in the genomes of the world's best athletes.
THE SPEED GENE
YANNIS PITSILADIS always keeps a few cotton swabs and Ziploc bags in his carry-on, just in case he runs into a world-record holder. In a decade of traveling to Ethiopia, Kenya and, more recently, Jamaica, Pitsiladis has learned that the concentration of elite track and field athletes in the three countries—sprinters in Jamaica and distance runners in Ethiopia and Kenya—is so high that he is liable to bump into one on a plane in Kingston or at a table in a coffee shop in Eldoret, Kenya.
Whenever he meets such athletes, Pitsiladis, a biologist at the University of Glasgow, entreats them to give him saliva or bits of the insides of their cheeks. Pitsiladis is collecting the DNA of champions to see what they are made of. In his lab he has stored the biological blueprints of nearly a thousand elite long-distance runners and sprinters, among them many African world champions and world-record setters, both living and dead. Pitsiladis has begun scanning these genomes for performance genes, so that humanity might know the slate upon which nurture can etch greatness.
Scientists have linked more than 200 genes—albeit tenuously in most cases—to physical performance. Take ACTN3, the so-called speed gene, one of the most thoroughly studied performance genes. In 2003 a seven-scientist team published a study in The American Journal of Human Genetics in which 429 elite Australian athletes were tested for the ACTN3 gene. All people have two copies of that gene, each of which comes in one of two variants, R or X. The R variant instructs the body to produce alpha-actinin-3, a protein found only in fast-twitch muscle fibers, the kind that contract rapidly and violently to facilitate explosive movement, while the X variant prevents the protein from being created.
Eighteen percent of the "normal" people used as a control group had two X copies, and thus none of the power protein in their muscles. But not a single one of the 32 Olympic sprinters in the study had two X variants. Similarly, of the sprinters whom Pitsiladis has analyzed so far, not one current or former world-record holder has two X variants.
Such findings quickly transcended mere chatter at an academics' cocktail party: In the wake of the '03 study, the Manly Warringah Sea Eagles of Australia's National Rugby League began testing players so that workouts could be tailored for those apparently more disposed to explosive powerlifting and sprinting; and for $169 Atlas Sports Genetics in Boulder, Colo., will test your child for ACTN3 so that you can decide early on whether to steer the tot toward sprints or endurance sports. And it goes beyond ACTN3: The Duke football team, for instance, sought institutional permission to submit players' DNA to a university researcher who will look for genes that predispose players to particular injuries.
Studies that assess genes with an eye toward differentiating Joe Frazier from Joe Montana and Joe Mauer from Joe Sixpack now come out regularly, identifying stretches of DNA that appear to influence the power of someone's grip, for example, or the strength of his ACL. Genes have been implicated not only in how much better an individual's aerobic capacity will get with regular bicycle training—the answer for some people, surprisingly, is "not at all"—but also in how likely he is to get off the couch in the first place.
Still, as more and more genes with subtle effects on athleticism have been identified, the predictive value of any single-gene test for talent, such as the ACTN3 tests offered commercially, has fallen essentially to nil. In 2008 Pitsiladis tested Colin Jackson, a British former world-record holder in the 110-meter hurdles, for ACTN3. "He had the right version," Pitsiladis says, "but so do I." And so do billions of people worldwide, but that doesn't make them world-class sprinters. In fact, it seems that all an ACTN3 test can do consistently is tell someone he isn't going to make the Olympic 4 √ó 100 relay team. But even that sweeping conclusion leaves room for exceptions, such as the Jamaican sprinter who was recently found to have the "wrong" copies of the explosiveness gene, or the Spanish long jumper who also has them yet sailed more than 27 feet and twice made the Olympics.
"He obviously still found a way to be pretty springy," says Carl Foster, director of the Human Performance Laboratory at Wisconsin--La Crosse and co-author of several ACTN3 studies. "If you want to know if your kid is going to be fast," Foster adds, "the best genetic test right now is a stopwatch. Take him to the playground and have him race the other kids."
The case of the curiously alpha-actinin-3-deficient long jumper means that running and jumping are influenced by a tangled skein of genes, not to mention training. So far, around two dozen genes have made strong scientific cases for inclusion in the equation of explosiveness, as have about two dozen for endurance.
What Pitsiladis is trying to do, in his words, is understand what makes the perfect athlete. He and colleagues have begun to sift through the genomes of some of his world beaters in hopes of finding one who, based on the performance genes identified thus far, is genetically perfect. He started with Jamaican sprinters and was startled by his preliminary findings. But before considering the genes that athletes want to have, let's consider one that they don't.
KNOWLEDGE EQUALS POWER?
ON A NOVEMBER afternoon in Manhattan, Ron Duguay, who played 12 seasons in the NHL, mostly as a center for the New York Rangers, was bracing for bad news. Duguay fiddled with his mammoth Rangers pinkie ring as he sat in a doctor's office overlooking Park Avenue South. Duguay was famous in the 1980s for playing without a helmet, and even at 52 the long brown tresses that made him a sex symbol have barely thinned.
He was in town to talk with Dr. Eric Braverman, hoping to get some help with his deteriorating memory. "I've had people tell me I should write a book about my career," Duguay said, "but I'd have to call up my teammates. There's a lot I can't remember." He guesses that he had between one and three serious concussions during a career that spanned from 1977 to '89, and he frequently took sticks or pucks to his unprotected head. After a battery of exams Braverman informed Duguay that he had flunked three tests of his memory and of the processing speed of his brain. "He's a mess compared to his old self," Braverman said. Braverman also ordered a test to see what versions Duguay has of the ApoE gene.
Duguay's grandmother died from Alzheimer's disease. Studies of Alzheimer's patients have found that a particular variant of the ApoE gene is associated with increased risk of the disease. Each person has two copies of the ApoE gene. (Each one is either ApoE2, ApoE3 or ApoE4.) People with one copy of ApoE4 have a three- to fourfold increased risk of Alzheimer's, while people with two ApoE4 copies have eight times the risk. Alzheimer's patients with ApoE4 variants also tend to show signs of dementia at an earlier age than Alzheimer's patients who do not have the variant.
The more ApoE has been studied, though, the more it has been associated not only with Alzheimer's but also with the ability of the brain to heal from all manner of trauma. People with ApoE4 variants who hit their heads in car accidents, for example, are more likely to have permanent damage or to die than those who have other variants. And a series of small studies suggests that athletes with ApoE4 variants who get hit in the head are more likely to recover slowly and to suffer greater dementia later in life. It is not entirely clear how ApoE affects brain recovery, but the gene is involved in the inflammatory response of the brain after injury, and people with the ApoE4 variant appear to take longer to clear their brains of a particular protein called amyloid, which floods in following head trauma.
A 1997 study of 30 boxers found that those who had taken a lot of blows to the head and had an ApoE4 copy scored significantly worse on tests of brain impairment than similar fighters who did not have an ApoE4 copy. The ApoE4 variant is present in less than 25% of the general population, but it was present in all three of the boxers in the study who were severely impaired. A 2000 study of 53 active pro football players concluded that three factors caused some players to score lower than others on tests of brain function: 1) age, 2) having been hit in the head a lot and 3) possessing an ApoE4 variant.
Last year, during the NFL's concussion controversy, doctors from Boston University made news with research on dozens of cases of brain damage in deceased football players and boxers. What escaped the news was the genetic data the researchers had for nine of the athletes. Five of them, or 56%, had at least one ApoE4 variant, more than twice the proportion found in the general population.
Two years after the study of the 53 football players, Barry Jordan, one of its authors and until a year ago the chief medical officer of the New York State Athletic Commission, considered requiring ApoE screening for all boxers in the state but then backed off. Doctors agree that more work is needed to understand how ApoE4 affects brain recovery before a genetic test should become common practice. Jordan and James P. Kelly, a neurologist on the Colorado State Boxing Commission, cited two other arguments against offering an ApoE test to athletes: first, teams and insurance companies might unfairly discriminate against an athlete with a certain gene; second, to tell someone he has an ApoE4 variant is to tell him about his risk of developing Alzheimer's later in life, information he might not want to know. "With ApoE4, some would argue that knowledge is not power," Kelly says.
Part of the concern over the insurance issue was allayed last year when the federal Genetic Information Nondiscrimination Act took effect, barring employers and insurance companies from discriminating on the basis of genetic information. And research at Boston University has found that people who volunteer for an ApoE screen do not feel undue dread if they find out they have the deleterious variant. In fact, they usually embrace lifestyle habits, such as exercise, that doctors tell them might decrease their Alzheimer's risk. "This is a very controversial area," says Robert C. Green, a BU neurologist who helped conduct the work. "The world of genetics for decades has suggested that there's no reason to give people genetic-risk information unless there's something proven you can do about it."
For athletes facing head trauma, perhaps there are some relatively painless actions they could take. "If this gene is how you're describing it," says Duguay, whose test showed that he has one ApoE4 copy, "and I knew I had it when I was playing, I would've seriously considered wearing a helmet."
Glen Johnson, a 41-year-old boxer with a 50-13-2 record, including wins over Roy Jones Jr. and Antonio Tarver, says he was considering retiring after his November loss to Chad Dawson. The older Johnson gets, the more he wants every bit of information that can tell him about what his life might be like after he quits fighting. Johnson—who understands that it isn't a particular gene variant, but rather getting hit in the head, that is the key factor in brain injury—has already put his relatives and friends on alert to tell him if they notice any differences in his speech or memory. "I'd have to get a better understanding of [ApoE4], and I'd take a lot of other things and tests into consideration when I think about fighting again," Johnson says, "but I'd never hide from extra information."
FINDING THE PERFECT ATHLETE
IF PITSILADIS IS to pinpoint the athletically perfect genetic specimen, he or she must first exist. Just how many of these folks might have stepped right off Mount Olympus is a question that kept Alun Williams awake two years ago. Williams, a geneticist at Manchester Metropolitan University in England, and a colleague pored over the scientific literature and chose the 23 genetic variants that have been most strongly associated with talent in endurance sports. The scientists gathered information about the variants' prevalence—some are found in more than 80% of people and others in fewer than 5%—and made statistical projections of how many "perfect" endurance athletes (people with two "correct" variants of each of the 23 genes) stride the earth.
Williams figured that perfection would be rare. After all, a Lance Armstrong comes around only once in a lifetime. But Williams was shocked when he ran the algorithm on his computer and saw that the odds of any person having all the right gene variants for endurance were less than one in a quadrillion. That's a one followed by 15 zeroes. Think of it this way: If you pony up for 20 tickets each week, you'd have a better chance of winning the Mega Millions twice in a row than of hitting this genetic jackpot.
The bottom line is that even Lance isn't a perfect specimen. Based on only the 23 chosen genes, there's almost certainly no genetically perfect athlete alive. In fact, given that a measly 6.8 billion people live on our planet, chances are that nobody has the ideal endurance profile for more than 16 of the 23 genes. An individual is also unlikely to have only a few of them. Essentially everybody falls in the muddled middle, differing by only a handful of genes. It's as if we've all played genetic roulette over and over, moving our chips around, winning sometimes and losing sometimes and gravitating toward mediocrity. "We're all relatively similar because we're relying on chance," Williams says.
But if anyone is the beneficiary of a long genetic winning streak, it should be a world-record holder, shouldn't it? Pitsiladis selected 24 gene variants most often associated with sprinting or endurance prowess and looked for them in the genomes of four men who have held the world record in the 100-meter dash and five who have held the world record in the marathon. What he saw was that based on those genes, the world-beaters are not genetic outliers at all. Pitsiladis analyzed the DNA of some of his graduate students for comparison and found that "a student of mine has a better rating for sprinting than the likes of an Asafa Powell or Usain Bolt." (Pitsiladis is legally prohibited from identifying specific athletes with their genetic material, so he used Powell and Bolt as rhetorical examples.)
That rather startling result leaves two broad possibilities: First, there is a tremendous amount of work left to be done to find all the remaining genes that contribute to athletic success; second, something other than genetics is at work. Both may well be true, but only time and more research will rule on the former, while Pitsiladis has compiled considerable data on the latter.
Some of the most intriguing work comes from his study of the demographics of elite East African distance runners. When Pitsiladis analyzed Kenyan runners, he found that three quarters of all elite international competitors were from a single tribe, the Kalenjin, who make up a mere 10% of Kenya's population. At first blush that would seem to indicate a genetic advantage in the Kalenjin, but Pitsiladis also found that they were likely to be living and training at altitude in the Rift Valley. When Pitsiladis compared 400 elite Kenyan athletes with a group of randomly selected Kenyans, he found that as children, the athletes were more likely to have lived at least several miles from school, and much more likely to have had to run there and back. Eighty-one percent of the elite Kenyan runners he studied had to rely on their feet to get to and from school, compared with only 22% of the control group. One 10-year-old boy whom Pitsiladis tested last year was already such an experienced runner that he clipped off six-minute miles when Pitsiladis tested him on a dirt track.
Haile Gebrselassie, the world-record holder in the marathon and perhaps the greatest distance runner ever, began running to school when he was five, covering more than six miles each way. For Ethiopians like him, Gebrselassie says, "every day is running. Every job is running: working in the fields or just getting somewhere. Life is running." (The statistics bring to mind a mock charity drive announced in the late 1990s on a now defunct online track and field message board: Help Americans compete in distance running by donating school buses to Kenyan children.)
Pitsiladis and his colleagues found a similar pattern in Ethiopia. What was not shared between the Ethiopians and the Kenyans, however, was a particularly large proportion of their genes.
The maternal line of DNA has been found to be more influential in endurance than the paternal, so Pitsiladis's group analyzed that genetic material and found that Ethiopian and Kenyan athletes "could not be more different genetically," Pitsiladis says. The Ethiopian athletes, for example, were much more likely to have blocks of gene variants common in Europe and Asia than were the Kenyan athletes. Pitsiladis's conclusion is that whatever specific genes are necessary for endurance, they aren't exclusive to either Ethiopians or Kenyans.
His work suggests that some sports phenomena that seem on the surface to be entirely based in genetics might not be. (Or at least not in the way we're used to thinking about genetics. A newer science called epigenetics is unraveling how environment and behavior, such as exercise, can actually turn particular genes on and off in patterns that might be passed on through generations.)
Similarly counterintuitive conclusions about the interplay between nature and nurture have come from outside sports: African-Americans are more prone than white Americans to hypertension, but the trend is not found among black people in some of the countries from which black Americans came, such as Jamaica and Nigeria. That points to the U.S., not to genes associated with blackness, as a culprit.
This is not to say that all ethnicities are the same. Nigerians are known for sprinting, and all Nigerians have at least one copy of the sprint gene variant. But so do nearly all Kenyans, as well as 80% of Europeans, two groups not renowned for sprinting.
A decade ago, when Pitsiladis began to study elite athletes, his medical students would ask why East Africans dominate distance running, to which he would reflexively respond that their secret is in their genes. "But after 10 years of work," he says, "I have to say that this is a socioeconomic phenomenon we're looking at." He feels the same way about sprinters in Jamaica, where scores of spike-clad kids hoping to be the next great runner attend sprint practice on Saturday mornings. (In the 1930s some U.S. sportswriters looked at the prominence of Jewish players in college basketball and suggested that Jews had a genetic predisposition for the game. That notion seems laughable today; the phenomenon was more likely due to the fact that inner-city New York, where many Jews lived then, was a basketball hotbed.)
Strong as the socioeconomic argument is, Pitsiladis adds, "This is not to say that genes aren't important. You absolutely must choose your parents correctly." That is facetious, of course, because we can't choose our parents. Nor do humans usually couple because of one another's genetics. We pair up more in the manner of the roulette ball that bounces off a few walls and eventually settles into one of many suitable spots. Williams suggests that if humanity is to produce an athlete with more of the "correct" sporting genes, the best approaches are to keep spinning the wheel (i.e., keep having babies) or to weight the genetic roulette ball with more lineages in which parents and grandparents have obvious athletic prowess and probably a lot of beneficial genes. The witting merger of superstars (think Yao Ming's parents, who were brought together by the Chinese basketball federation) is rare. Yet there is a particular population in which it is the standard.
THE NATURE OF NURTURE
IF THOROUGHBREDS COULD talk, they might explain just how important it is to choose one's parents. Because athletic ability involves a complex mix of genes, champion racehorses tend to result from multiple generations of mating among athletic horses. The more genes that are involved in an athletic trait, the more generations of athlete-to-athlete breeding it will likely take to get a colt that has collected enough of the right gene variants to make the winner's circle.
Thus the one sure bet at the racetrack is that every top horse has good racehorses not only for parents but also for grandparents and great-grandparents. (In some marquee horse races, winning times plateaued in the mid-20th century, leading some scientists to suggest that centuries of breeding for speed have in fact brought thoroughbreds to their physiological terminal velocity).
This phenomenon has also been demonstrated repeatedly with lab rats. When rats who can run farther than average before giving in to exhaustion are bred with one another, each generation has successively greater endurance.
Family lineage is critical for humans as well. Since 1992 the HERITAGE Family Study has analyzed hundreds of families to find out how "heritable" exercise capacity is, or how much variation in a particular fitness trait is due to genetic inheritance. In one segment of the study, 98 families that had not been exercising were put on identical 20-week training programs on stationary bikes. Their aerobic capacity—a gauge of how much oxygen the body can use during exercise—was measured before the program and at the end. In terms of improvement, family members tended to be similar, while improvement varied widely among families. Even after five months of training, some people in families that benefited little on average did not improve their aerobic capacity one iota, while others in families that generally showed marked improvement increased it up to 50%. Statistical analysis showed that about half of a person's ability to improve with training was determined by his or her parents. The amount any person improved in the study had nothing to do with how good he was to begin with—his "baseline aerobic capacity"—but about half of that baseline, too, was attributable to family inheritance.
In work from the HERITAGE study that has not yet been published, the researchers have identified 20 genetic markers that can predict entirely the genetic component of an individual subject's aerobic capacity improvement after months of bicycle training. The point of the work is to be able to give people individualized advice regarding whether exercise is the key to improving their health, or whether they are among the low responders who might be better off resorting to medication. There are also implications for athletes: These 20 genetic markers can help predict whose strong work ethic will pay off in aerobic spades and who might be pedaling largely in vain.
The HERITAGE Family Study is controlled science, with all subjects on identical training plans. In reality, though, one of the most hallowed notions in sports is that we can each carve our athletic destiny by waking up earlier and working out harder than the guy or girl at the next locker—that is, by adopting different training plans. But researchers have found that even motivation to work out has an important genetic component. A 2006 Swedish study of more than 13,000 sets of fraternal and identical twins—fraternal twins share half their genes on average, while identical twins share all of them—found that the exercise tendencies of identical twins were twice as likely to be similar as those of fraternal twins.
Another 2006 study, of more than 37,000 pairs of adult fraternal and identical twins from six European countries and Australia, concluded that about half to three quarters of the variation in the amount of exercise people engaged in could be accounted for by their genetic makeup, while environmental factors, such as access to a gym, often had less influence. And studies of laboratory mice suggest that the difference could be in genes that regulate dopamine, a brain chemical involved in sensations of pleasure and reward.
For more than a decade Theodore Garland, a physiologist at UC Riverside, has been giving mice a wheel and allowing them to hop on or spurn it. Normal mice voluntarily run three to four miles each day, but Garland and his colleagues separated the mice that chose to run the farthest and bred them with each other. By generation 16 the high runners were pounding out more than seven miles a day. "The normal mice are out for a leisurely stroll," Garland says. "They putz around on the wheel, while the high runners are really running."
But one interesting difference was that the high runners' brains were larger. "Presumably, the centers of the brain that deal with motivation and reward have gotten larger," Garland says. His team plied mice with Ritalin, a stimulant that alters dopamine levels. Once doped, the normal mice, apparently gleaning a greater sensation of pleasure from activity, suddenly ran more. But the doped long runners did not. Whatever Ritalin does in the brains of normal mice is already happening in the brains of the high runners. They are running junkies.
Other studies have begun to identify the specific genes related to dopamine processing that differ between the marathon mice and their average counterparts. "Who says motivation isn't genetic?" Garland asks rhetorically. "In these mice it's absolutely the case that motivation has evolved." A 2003 study found an association in women between physical activity and a particular dopamine receptor gene, DRD2.
Wayne Gretzky famously said, "Maybe it wasn't talent the Lord gave me, maybe it was the passion." But what if the two are inextricable? What if passion is a talent? Might not, say, Floyd Mayweather Jr.—who has been known to jolt awake in the middle of the night salivating to get to the gym—get an innately outsized sense of reward from working out? This gets to the questions that genetics has brought us no closer to answering: Where does genetic motivation bleed into free will? How can we affect our athletic fate? Who's in charge here—is your body running you, or are you running it?
After Mayweather's win over Oscar De La Hoya in 2007, he told SI about an unhappy period in the past when he constantly worried about money. "But I'm happy now," he said giddily, mentioning the $25 million he had made from the fight. Certainly, rewards more obvious than dopamine—such as money—are huge motivators. And, as it does with so many human endeavors, money can counter athletic heritage. "How many of the top Kenyan runners have sons or daughters who are excelling at running?" asks Pitsiladis. "Almost none. Why? Because their father or mother becomes a world champion, has incredible resources, and the child never has to run to school again."
The study of sports genes has identified specific genes, such as ACTN3, that help explain why some people will probably never run a 4.3 in the 40. Genetic research has also suggested that some athletic phenomena that appear to be entirely genetic may have critical environmental factors, while others that appear to be entirely feats of will may have important genetic components. Scientists are rapidly identifying more performance genes and working to tease apart their subtle influences.
In terms of coaching tips, however, the scientific work has yielded little practical advice beyond what we already knew: Each athlete is an experiment of one, sports are good for all of us, some of us might be more motivated to practice than others, and the only way to find out how good each of us can be is to cross our fingers and train. Even though genes are critically important to an individual's athletic potential, at this point in sports-science history we are left in the position of Greek tragic heroes: circumscribed by nature, but with ill-understood wiggle room to direct our fates.
This may change, of course, as scientists now know that the path between genes and behavior is a two-way street. One study found that when people habitually lifted weights, a gene that codes for a muscle-growth-inhibiting protein became less active. And scientists studying epigenetics are beginning to learn how our behavior might alter the expression of our children's genes.
In the near future the study of genetics promises to tell us more about who we are as individual athletes, how much we can change and how best to go about it. "Genes do not act in a vacuum," notes Bernd Heinrich, a biologist and author and the 1981 North American 100K champion. Heinrich, who grew up running to school, insists that "genes are very plastic. They can be turned on or off. Look at a caterpillar and a butterfly: They've got the same genes. One flies, and one can barely crawl."
NEXT UP: GENE DOPING
ADVANCES IN GENE THERAPY TO HELP COMBAT DISEASE HAVE ATTRACTED THE INTEREST OF ATHLETES—AND OF SPORTS ANTIDOPING AUTHORITIES
As medicine enters the era of gene therapy, some athletes will find performance-enhancing applications in it—if they haven't already done so. Gene therapy treats patients by inserting synthetic genes, called transgenes, into their bodies, generally by handing the genes off to a virus that carries it into cells.
In his search for treatments for muscle-wasting diseases, Se-Jin Lee, a professor of molecular biology and genetics at Johns Hopkins's medical school, created mice with twice the amount of muscle of normal rodents by deactivating a gene that codes for myostatin, a protein that tells muscles to stop growing. When Lee published his results in 1997, he received e-mails not only from muscular dystrophy patients but also from bodybuilders and other athletes wondering if their genes could be altered to make them like the mighty mice.
Transgenes could have athletic applications as well. The PPAR-delta gene helps determine how much energy-producing mitochondria is in a muscle cell and thus how fatigue-resistant the muscle is. In 2004 scientists tweaked PPAR-delta in rodents to produce "marathon mice," which ran 67% longer and 92% farther than normal mice.
A transgene for the hormone erythropoietin, the EPO of Tour de France infamy, promotes production of oxygen-carrying red blood cells and has been tested in primates. The gene worked too well in baboons, which had to be bled in order to survive after their bodies overproduced red blood cells, which dangerously thickened their blood. In some macaques the gene caused an autoimmune response that interfered with EPO function in their bodies, leaving them severely anemic.
Tantalizing success and catastrophic failure has also characterized human gene-therapy testing. A gene-therapy trial in France was suspended in 2004 after three of nine boys who were cured of X-linked severe combined immunodeficiency—a.k.a. Bubble Boy syndrome—developed leukemia. Nevertheless, scientists are learning how to make gene therapy safer: In trials gene therapy has improved the condition of patients with skin cancer and retinal disease.
Meanwhile, antidoping researchers are pushing to get ahead of the gene-doping curve. The World Anti-Doping Agency has funded research to develop methods of detecting gene doping by measuring the response of other genes to a transgene. But could cheating athletes already be ahead of the testers? At a panel of sports doctors in Vancouver in March, Jim Rupert, an associate professor at the University of British Columbia, said, "Gene doping may already be a trend. Nobody is really sure."
There have not been any clear-cut cases, but there have been worrisome harbingers. In the 2006 trial of German track coach Thomas Springstein for providing performance-enhancing drugs to minors, it was revealed that Springstein had tried, probably without success, to procure the experimental EPO-boosting gene-therapy drug Repoxygen.
THE UNCOMMON THREAD
IN A FEW CASES A SINGLE GENETIC MUTATION HAS BEEN DIRECTLY LINKED TO HEIGHTENED ATHLETICISM
Stephen M. Roth, an associate professor of kinesiology at Maryland, is among a group of scientists who track all the published work on potential performance genes and compiled a list known as the Human Gene Map for Performance. Roth notes that even seemingly straightforward athletic attributes, such as raw strength, are highly complex, influenced as they are by multiple genetic and environmental factors. "With any single gene that seems to have a positive effect on performance, the effect is very subtle," Roth says. There are, however, a few documented cases in which a single, rare genetic mutation has hugely increased an individual's athleticism.
• Eero M√§ntyranta is a former cross-country skier who won three gold, two silver and two bronze medals for Finland in three winter Olympics in the 1960s. But suspicions of blood doping cast a pall over his achievements, because he had 20% more oxygen-carrying red blood cells than his competitors despite his claim that his training was no different from theirs. Three decades later, in 1993, Finnish scientists published the results of a study that examined five generations of his family. The study found that a rare mutation on the EPOR gene runs in the M√§ntyranta family, causing an excessive response to erythropoietin, or EPO, a hormone that spurs red-blood-cell production. In essence, M√§ntyranta and 28 relatives who were studied have naturally what some athletes gain only by cheating with EPO injections. Not surprisingly, the family has a number of accomplished endurance athletes.
• In 2000 a German doctor was startled by the leg-muscle definition of a newborn boy at the Charité hospital in Berlin. Four years later this so-called superbaby had calves and glutes far more developed than those of his peers, and he could hold a pair of 6.6-pound dumbbells at arm's length. Further study determined that a rare mutation on two genes prevents the child from producing myostatin, a protein that helps tell muscles when to stop growing. The boy's mother has the same mutation on one of her two myostatin genes, leaving her with some myostatin but less than an average person has. She is the only adult with a documented myostatin mutation to date, and she was a professional sprinter.
• In 1969 a quarter horse named Impressive was born with a mutation on a gene that affects the flow of sodium into muscle cells. That allowed Impressive's muscles to contract both more quickly and more fully than those of other quarter horses, and in 1974 it helped him win the Open Aged world championship, a first for his breed. Impressive was intensely sought for breeding, and he sired dozens of world champions.
Such rare gene mutations have downsides, however. M√§ntyranta's condition can cause excess strain on the heart. As for the German superbaby, doctors can only wonder whether his condition will cause his muscles, including the heart, to grow bigger than is healthy. And Impressive's mutation can cause a disease called hyperkalemic periodic paralysis, which is potentially fatal. Because Impressive spread the mutation to so many horses, his condition is sometimes known as Impressive syndrome.
THE SINS OF THE FATHER . . .
LISTEN UP, COUCH POTATOES: ACCORDING TO THE SCIENCE OF EPIGENETICS, HOW PARENTS ACT INFLUENCES THE BEHAVIOR OF THE GENES THEIR CHILDREN INHERIT
You had the talent to be a sports star, but you lacked the discipline. You couldn't bring yourself to go to bed early or pick up a barbell instead of an Oreo. On the bright side, your kid gets to start from square one: He or she inherits your talent but not the repercussions of your lackluster approach.
Or maybe not. Research in the growing science of epigenetics—which, among other things, looks at how genes can be switched on and off—suggests that your actions could have genetics-related consequences for your child. Better think twice before pounding down that next cookie.
At the turn of the 19th century, French naturalist Jean-Baptiste Lamarck proposed a theory of evolution that said animals quickly adapt to their environment and pass the adaptations along to their progeny; for example, giraffes acquired long necks because their ancestors stretched to reach leaves high on trees. But Lamarckian evolution was eclipsed in 1859 when Charles Darwin published his theory of evolution by natural selection, which says traits are acquired over millions of years as random genetic changes that happen to be beneficial are passed on. Today, however, the idea at the heart of Lamarck's theory—that our choices can affect our children's genes—is making a comeback.
Your behavior will not change the sequence of your child's DNA, but it might change the action of certain molecules—called epigenetic marks—that attach to DNA and signal genes to turn on and off. Consider a study, published in 2008, of adults in the Netherlands whose mothers had suffered through a German-imposed food embargo in the winter of 1944--45. Six decades later, those whose mothers were in the early stages of pregnancy during the Dutch Hunger Winter had fewer "turn off" signaling methyl molecules attached to their IGF2 genes than did their siblings. The IGF2 gene is a key component in growth and development, and the study may help explain why children of mothers who experience famine during pregnancy have a higher risk of developing obesity, schizophrenia and diabetes later in life.
Studies now under way should further illuminate the epigenetic link to sports prowess. In one study published last year, rats that exercised regularly and rats that didn't were twice thrown into beakers of water. On the first go-round, all the rats struggled vigorously to swim and attempt to escape the beaker. A day later, when they were again put into the water, the regularly exercised rats displayed better stress-coping mechanisms: Instead of clawing at a glass wall they could not climb, these rats, having learned from the previous day's experience, conserved energy by floating. When their brains were examined afterward, researchers found that the exercised rats had altered epigenetic marks that in turn affected gene expression in a part of the brain that helps form memories. "Exercise has a great impact on the brain, not just the muscles," says Johannes Reul, one of the researchers and a neuroscientist at the University of Bristol, England.
Perhaps one day a similar epigenetic explanation of memory will help us understand why great cornerbacks don't get beat the same way twice.
MAKINGS OF AN ATHLETE
MORE THAN 200 GENES HAVE BEEN LINKED TO PERFORMANCE, AFFECTING EVERYTHING FROM EXPLOSIVENESS TO THE STRENGTH OF LIGAMENTS. HERE ARE JUST A FEW
GENE
DRD2
BRAIN
PHYSICAL ACTIVITY LEVEL
GENE
ADRA2B
SKELETAL MUSCLE
FAT METABOLISM AND RESTING HEART RATE
GENE
ATP1A2
LUNGS
IMPROVEMENT OF AEROBIC CAPACITY WITH TRAINING
GENE
BDKRB2
QUADRICEPS
QUAD STRENGTH AND EFFICIENCY OF MUSCLE CONTRACTION
GENE
PPARA
HEART
GROWTH OF LEFT VENTRICLE WITH TRAINING
GENE
VDR
BONES
GRIP STRENGTH AND BONE DENSITY
GENE
EPAS1
BLOOD VESSELS
RED BLOOD CELLS THAT CARRY OXYGEN TO MUSCLES
GENE
ACE
LEGS
VERTICAL JUMP AND AEROBIC ENDURANCE
GENE
ACTN3
FAST-TWITCH MUSCLES
SPRINT SPEED AND POWER
FOUR ILLUSTRATIONS
ILLUSTRATIONS BY BRYAN CHRISTIE
PHOTO
AP
PHOTO
NEW ENGLAND JOURNAL OF MEDICINE/AP
PHOTO
DON TROUT